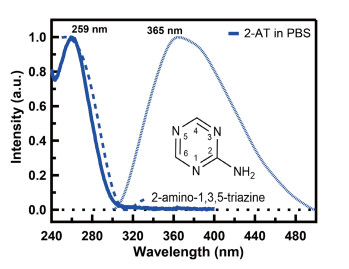
Citation: | Xiuhua Li, Xueli Wang, Meng Lv, Zhongneng Zhou, Haifeng Pan, Jinquan Chen. Direct Observation of Singlet ππ* and nπ* Equilibrium State in 2-Amino-1,3,5-triazine Solution[J]. Chinese Journal of Chemical Physics , 2022, 35(5): 747-753. DOI: 10.1063/1674-0068/cjcp2202029 |
1,3,5-Triazine molecules with functionalized group on the ring can mimic nucleobases (both pyrimidine and purine) and form duplex hybrids in DNA or RNA as well as in some self-assembly super-molecules [1, 2]. Moreover, it has been shown that 1,3,5-triazine molecules (hereafter, named triazines) can be synthesized from urea in ice matrix under reductive atmosphere condition [3]. Therefore, triazines are envisaged to be the primordial nucleobase alternatives, which may be the building blocks for the primitive informational polymers that preceded RNA world. Since it is well known that the solar UV flux down to wavelengths as short as 200 nm was significantly higher on early Earth's surface due to the lack of ozone [4], the intrinsic photo-stability against UV radiation is one of the key properties that determine whether the molecule could become the primitive informational carrier [5, 6]. For example, the canonical nucleobases, which are the genetic information carriers for most of life on Earth, have been proven to have high UV hardiness because of their sub-picosecond excited state lifetime [7−9], minimizing the possibility of chemical reactions such as photoionization, photodissociation, ring-opening, as well as electron and energy transfer between adjacent molecule and solvent.
The UV hardiness of triazines was often overlooked in previous studies until some femtosecond transient absorption studies occurred in recent years. Brister et al. reported that 2, 4, 6-triaminopyrimidine has a sub-picosecond 1ππ∗ state lifetime and a 1nπ∗ state lifetime of several ps in aqueous solution [10], which suggests it should have high UV hardiness. Zhang et al. showed that both melamine (2, 4, 6-triamino-1,3,5-triazine) and its lysine derivative have a ∼10 ps dominant deactivation pathway to dissipate their excited state population. Yet, a long-lived triplet state is proposed in these compounds. Previously, we studied 2-amino-1,3,5-triazine (2-AT) in aqueous solution and discovered a 2.6 ps component and another longer-lived component (>100 ps). Due to the detection limit at that time, the origin of the longer-lived component has never be revealed. In this work, we report the full picture of excited state dynamics of 2-AT in different solvents. Interestingly, the previously observed longer-lived component is demonstrated to be an equilibrium state consisting of the almost energy degenerated bright ππ∗ and dark nπ∗ states in 2-AT in phosphate buffer solution (PBS) at pH=7.4. Moreover, this state could further decay by fluorescence emission, internal conversion, or intersystem crossing to triplet state and it is possible to alter the excited state decay pathways through different solvent environment. This is one of the few cases that an equilibrium state can be directly observed in small organic molecules and the solvent sensitive excited state dynamics provides valuable information for further designing potential environmental sensitive applications of 2-AT.
2-AT, acetonitrile (99.9%) (MeCN), methanol (MeOH) were purchased from J & K Chemical Ltd. (Shanghai, China) and used as received. Tetrahydrofuran (THF) was purchased from Aladdin Chemical Technology Co. Ltd. (Shanghai, China).
Steady state UV-Vis absorption spectra and fluorescence emission spectra were measured with a commercial spectrometer (TU1901, Beijing Purkinje General Instrument Co. Ltd.) and a fluometer (FluoroMax-4, Horiba, Jobin Yvon) at room temperature, respectively.
The transient absorption (TA) setup was reported before [11]. Briefly, a femtosecond laser system (Astralla, Coherent Inc.) was used to generate the 800 nm fundamental beam. The pump pulse was generated by an optical parameter amplifier (OPerA Solo, Coherent Inc.). The probe pulse was produced in a commercial TA spectrometer (Helios-EOS fire, Ultrafast System). Polarization between pump and probe beams was set to be 54.7∘. The instrumental response was determined to be ∼120 fs. Nanosecond TA spectra were also measured with the commercial TA spectrometer (EOS, Ultrafast Systems) [12].
Femtosecond fluorescence up-conversion spectra were collected using a home-built fluorescence up-conversion setup. The detailed information about the experimental setup has been reported elsewhere [13]. In brief, the pump pulse was the same as the femtosecond TA setup and it was spectrally filtered by a pair of UV fused-silica prisms. A small portion of the fundamental light was used as the gate pulse. A pair of parabolic focus mirrors was applied to collect fluorescence and then focused into a 0.5 mm β-BaB2O4 (BBO) crystal. The up-conversion signals were collected with a monochromator (Omnik500, Zolix) and detected using a photomultiplier tube (CR317, Hamamatsu). The time resolution was determined to be ∼400 fs.
Triplet state quantum yield of 2-AT was measured in water using the trivalent europium ion (Eu3+) method described in Ref.[14]. The intensity of the luminescence of Eu3+ (monitored at 590 nm) in H2O sensitized by 2-AT and 6-azauracil (whose triplet quantum yield was measured to be unity) was measured as a function of the Eu3+ concentration. The donor concentration was about 1 mmol/L at all cases. The pH was kept below 5.0 to ensure that no precipitation or association of the Eu3+ with the phosphate groups took place. The excitation wavelength was 267 nm.
The ground state structures of 2-AT in different solvents were optimized using time-dependent density functional theory (TDDFT) [15] with B3LYP [16] functional and 6-311++G(d, p) basis set [17]. The vertical excitation energies were also computed by TDDFT calculations. Truhlar's universal solvation model (SMD) [18] was employed to take into account of the solvent effects.
FIG. 1 depicts the experimental UV/Vis absorption and fluorescence emission spectra of 2-AT in PBS. The pKa value of 2-AT has been reported to be 2.9 and 14.9 [19], suggesting that 2-AT is neutral form in buffer solution. The absorption maximum of 2-AT centers at 259 nm in PBS and the fluorescence emission peak of 2-AT is centered at 365 nm. TDDFT calculation results (detail below) suggest that the lowest energy absorption and emission should correspond to a bright ππ∗ state (S3) arising from HOMO→LUMO transition localized on the six-membered ring. The steady state absorption and emission spectra of 2-AT in MeOH, MeCN, and THF are also measured, and the results are displayed in FIG. S1 in Supplementary materials (SM). The absorption band shows a continuous red-shift as solvent polarity decreases from PBS to THF. However, the largest Stokes shift (∼1.5 eV) is observed in MeOH. Fluorescence emission is not measured in THF due to strong solvent background.
Previously, Zhou et al. reported that the lowest three singlet states of 2-AT had very close energy levels and the energy surface of the ππ∗ state could cross with those of the two nπ states almost in the Franck-Condon (FC) region, implying fast transition between ππ∗ and nπ∗ states could happen [11]. In this work, similar results were obtained from TDDFT calculations on 2-AT in water (Table Ⅰ). Since the oscillator strength of the ππ∗ (S3, 4.873 eV) state is the largest, it is expected to be primarily populated upon the 267 nm excitation. And there are two low-lying nπ∗ states (S2 and S1) with energies of 4.860 eV and 4.772 eV in 2-AT, respectively. It is worth noting that four low-lying triplet states were also documented. Among that, T4 has nπ∗ character and the energy of such a state (4.630 eV) is very close to that for low-lying singlet state, while T3−T1 has energies range from 4.330 eV to 4.129 eV.
![]() |
In addition, the TDDFT calculations on 2-AT were also carried out in MeOH, MeCN and THF solvents. The ground state (S0) equilibrium structures (FIG. S2 in SM) and first bright excited state (S3) structures of 2-AT are optimized and shown in FIG. S3 (in SM). The corresponding energies of the lowest four singlet and triplet states are summarized in Table Ⅰ. It is clear that the two nπ∗ states (S2 and S1) have almost degenerated energy with the bright ππ∗ state (S3). Compared with values in water, the energies of the lowest four singlet and triplet states only show slight difference in both MeCN and MeOH. In THF, both nπ∗ states have lower energies compared with those in water while the bright ππ∗ state shows higher energy. Nevertheless, the energy difference between the bright ππ∗ state and the two nπ∗ states is only ∼0.1 eV, which is beyond the precision of TDDFT and suggests that these three states could actually have energy degeneracy (FIG. S3 in SM).
Femtosecond time-resolved fluorescence up-conversion spectra were measured on neutral 2-AT in PBS and the results are displayed in FIG. 2. The kinetics can be globally fitted by two time constants for the detection wavelength from 320 nm to 380 nm (Table Ⅱ). The first lifetime (τ1) is 0.68±0.10 ps and the second lifetime (τ2) is 396±7 ps. It is also clear that the amplitude of τ1 keeps decreasing while that of τ2 keeps increasing as the probe wavelength shifts to the red side. These results indicate that there are two emissive species in 2-AT, which is not consistent with the TDDFT calculation that there is only one bright ππ∗ state. Detailed assignment of these two species is discussed below.
![]() |
Femtosecond TA spectra of 2-AT were measured in different solutions and the results are shown in FIG. 3. The excitation wavelength is 267 nm and it should directly populate the bright ππ∗ state (S3). In PBS, a broad excited state absorption (ESA) band with one peak at 350 nm and a shoulder peak at 450 nm shows up right after excitation. The shoulder peak quickly decays away in the first ∼10 ps while the main peak at 350 nm shows barely any difference. In the next ∼2 ns, the ESA peak at 350 nm gradually decays while a new ESA band with peak at 600 nm appears. Then, the whole TA spectra show no change during our 8 ns detection window. The early evolution of the TA spectra matches well with our previous report [11]. The evolution of TA spectra of 2-AT in MeCN is similar to that in PBS, but faster kinetics is seen. In MeOH and THF, the 450 nm shoulder peak seen in PBS is missing and the amplitude of the long-lived component is smaller. Nanosecond TA experiments were further carried out on 2-AT in MeCN and the spectra are shown in FIG. S4 in SM. The oxygen sensitive kinetics (FIG. S4 in SM) demonstrates that the long-lived component should originate from a triplet state. Triplet quantum yield of 2-AT in PBS is measured to be 47%±10% (FIG. S5 in SM).
Global and target analysis was applied on the TA spectra by using GloTarAn program [20]. Best fitting parameters are listed in Table Ⅲ and representative kinetics are displayed in FIG. S6 in SM. Decay associated spectra (DAS) are extracted and shown in FIG. 3. In PBS, the first DAS (yellow) has lifetime of 2.5 ps and shows the ESA peak at 450 nm along with a dip around 350 nm. This corresponds to the decay of the shoulder band seen in the first ∼10 ps. The second DAS (purple) shows the main peak at 350 nm and its lifetime is 522 ps. Negative amplitude from 450−650 nm indicates that the ESA signal in this region increases during this lifetime. At last, the third DAS (dark green) is the long-lived component which has already been assigned to the triplet state. In MeCN, the spectral shape of DAS is similar to that in PBS except the lifetime. However, the first DAS is missing when solvent is turned into MeOH and THF. Moreover, the amplitude of the triplet state also decreases in these two solvents and it can only barely be resolved in THF.
![]() |
According to our previous and current calculations, the first bright state in 2-AT is the S3 state, which has a ππ∗ character and corresponds to the HOMO→LUMO transition localized on the six-membered ring [11]. However, there are two lifetimes determined in the fluorescence up-conversion spectra. For the first component, the lifetime is 0.68 ps. This could arise from a short-lived bright state or solvation of the final emissive state. After carefully checking the wavelength dependent kinetics, we exclude solvation for this component because we do not observe the decay in the blue side while build-up in the red side [21]. Moreover, the first DAS of 2-AT in PBS shows a small dip around 350 nm while more clear negative amplitude is seen in MeCN (FIG. 3), which is believed to arise from stimulated emission (SE). These results are in line with the fluorescence up-conversion data and it indicates that there is a short-lived emissive state in 2-AT. In our previous study, we have already shown that the bright ππ∗ state (S3) could cross with two nπ∗ states (S2 and S1) almost in the FC region by analyzing the minimum energy path from FC geometry to ππ∗min, suggesting the bright ππ∗ state of 2-AT should be short-lived [11]. Thus, we assign the 0.68 ps component (2.5/1.8 ps component in the TA spectra of 2-AT in PBS/MeCN) in the fluorescence up-conversion spectra to the bright ππ∗ (S3) state with confidence.
Now, we have to answer the question what is the origin of the second component with hundreds of ps lifetime observed in both fluorescence up-conversion and TA spectra of 2-AT. From the DAS, it is clear that the component with hundreds of ps lifetime is presented right after excitation in all solvents and it can evolve to the long-lived state, which is a triplet state. As mentioned above, the bright ππ∗ state (S3) could cross with two nπ∗ states (S2 and S1) in the FC region. Therefore, it is possible that the component with hundreds of ps lifetime could arise from one of the nπ∗ state in 2-AT. It has been reported that the nπ∗ state is the door way state to the triplet state in pyrimidines and its analogs [22−25], the lifetimes reported for these dark nπ∗ state are somehow similar to what we seen in 2-AT. However, our calculation has shown that the oscillator strengths of both nπ∗ states are much smaller compared with that of the bright ππ∗ state, suggesting that emission of these two states should be much weaker. Yet, the amplitude determined for the second component in the red side of the fluorescence up-conversion spectra can reach about half of that of the bright state, suggesting that the emission strength of this component should be comparable to the bright ππ∗ state. This result does not support the assignment of this component to the dark nπ∗ state because these states usually carry no oscillator strength in molecules with similar structure to 2-AT [26]. It seems that the component with hundreds of ps lifetime in 2-AT should have both nπ∗ and ππ∗ characters.
Recently, an equilibrium state with both singlet ππ∗ and triplet nπ∗ characters is discovered in ketones with xanthone structure (xanthone, thioxanthone, and acridone) [27−32]. Theoretical calculation reveal that there are four close-lying singlet and triplet ππ∗ and nπ∗ states in these ketones. In vacuum or apolar solvents, the 1nπ∗ state is the lowest excited singlet state and the corresponding 3nπ∗ state generally has a higher energy than the lowest 3ππ∗ state. When solvent polarity increases, the energy of the 1nπ∗ state is blue-shifted and the 1ππ∗ state becomes the lowest singlet excited state. If energy of the 1ππ∗ state is isoenergetic with that of the 3nπ∗ state, a singlet-triplet equilibrium state can be accessed due to fast intersystem crossing (ISC) and reverse intersystem crossing (rISC). The equilibrium state has several to tens of ps lifetime and it can further emit fluorescence or decay to the lowest 3ππ∗ state depending on the environment. Considering the almost degenerated energies of the lowest three singlet states in 2-AT (Table Ⅰ), we envisage that the component with hundreds of ps lifetime could originate from an equilibrium state between the bright ππ∗ state (S3) and the two nπ∗ states (S2 and S1). This assignment is not only supported by our calculation but also can well explain the relatively strong emissive strength as well as its excited state dynamics in PBS.
Since the ππ∗ and nπ∗ states generally have different response to solvent environment, the excited state dynamics of the equilibrium state is expected to be solvent sensitive. Solvent dependent TA experiments are carried out on 2-AT in order to further support the assignment of the equilibrium state. In MeCN, whose polarity is smaller than water, similar TA spectra and spectra evolution are observed but the lifetimes of the first two components are smaller than those in PBS (FIG. 3). The measured fluorescence quantum yield of 2-AT (Table S1 in SM), is smaller in MeCN, which is in line with the shorter lifetimes. It is known that the energy of the nπ∗ state is red-shifted in general when the solvent polarity is smaller [30]. In this case, the equilibrium state could break and excited state population could be trapped in the nπ∗ state. This is in line with the shorter lifetime of the equilibrium state in MeCN as it should exhibit more of its nπ∗ character (non-radiative decay to the ground state or ISC to triplet states). In MeOH, the component with several ps lifetime is missing, suggesting that internal conversion of the bright ππ∗ to the equilibrium state may take place faster than our instrumental response. It is worth pointing out that the SMD model used in our TDDFT calculation neglects the effect of hydrogen bonding, leading to overestimation of the nπ∗ energy level in MeOH. Since the nπ∗ character should dominate now, the fluorescence quantum yield further decreases (Table S1 in SM). At last, the energy gap between the ππ∗ and nπ∗ states is further increased when 2-AT is in THF (FIG. S3(d) in SM). In this case, the equilibrium state no longer exists and major excited state population should be trapped in the lower nπ∗ state (S1). The main decay pathway now is internal conversion to the ground state since the amplitude of the triplet state is tiny.
In conclusion, the excited state dynamics of 2-AT in different solvents are studied using time-resolved transient absorption and fluorescence spectroscopy. The excited state deactivation mechanism of 2-AT in PBS is proposed in Scheme 1. The most interesting discovery is that the initially populated bright ππ∗ state of 2-AT in PBS can decay to an equilibrium state formed by the almost energy degenerated ππ∗ and nπ∗ states. This state has 500 ps lifetime in PBS and it could further decay through fluorescence emission, ISC to the triplet state and internal conversion to the ground state. The excited state dynamics of this state is solvent sensitive as the equilibrium could break due to solvent polarity or hydrogen bonding. Our results provide a rare example in which a singlet ππ∗-nπ∗ equilibrium state can be directly observed in solution and provide valuable information for further applications of 2-AT.
Supplementary materials: Additional steady-state and time-resolved spectroscopy data are available.
This work was supported by Shanghai Rising-Star Program (No.19QA1402800).
[1] |
G. K. Mittapalli, K. R. Reddy, H. Xiong, O. Munoz, B. Han, F. De Riccardis, R. Krishnamurthy, and A. Eschenmoser, Angew. Chem. Int. Ed. 46, 2470 (2007). doi: 10.1002/anie.200603207
|
[2] |
C. T. Seto and G. M. Whitesides, J. Am. Chem. Soc. 112, 6409 (1990). doi: 10.1021/ja00173a046
|
[3] |
C. Menor-Salvan, M. Ruiz-Bermejo, S. Osuna-Esteban, and S. Veintemillas-Verdaguer, Origins Life Evol. Biospheres 39, 250 (2009).
|
[4] |
C. S. Cockell and G. Horneck, Photochem. Photobiol. 73, 447 (2001). doi: 10.1562/0031-8655(2001)073<0447:THOTUR>2.0.CO;2
|
[5] |
A. A. Beckstead, Y. Zhang, M. S. De Vries, and B. Kohler, Phys. Chem. Chem. Phys. 18, 24228 (2016). doi: 10.1039/C6CP04230A
|
[6] |
Y. Y. Zhang, A. A. Beckstead, Y. S. Hu, X. J. Piao, D. Bong, and B. Kohler, Molecules 21, 1645 (2016). doi: 10.3390/molecules21121645
|
[7] |
J. M. L. Pecourt, J. Peon, and B. Kohler, J. Am. Chem. Soc. 123, 10370 (2001). doi: 10.1021/ja0161453
|
[8] |
C. E. Crespo-Hernández, B. Cohen, P. M. Hare, and B. Kohler, Chem. Rev. 104, 1977 (2004). doi: 10.1021/cr0206770
|
[9] |
C. T. Middleton, K. De La Harpe, C. Su, Y. K. Law, C. E. Crespo-Hernández, and B. Kohler, Annu. Rev. Phys. Chem. 60, 217 (2009). doi: 10.1146/annurev.physchem.59.032607.093719
|
[10] |
M. M. Brister, M. Pollum, and C. E. Crespo-Hernández, Phys. Chem. Chem. Phys. 18, 20097 (2016). doi: 10.1039/C6CP00639F
|
[11] |
Z. Zhou, X. Zhou, X. Wang, B. Jiang, Y. Li, J. Chen, and J. Xu, J. Phys. Chem. A 121, 2780 (2017). doi: 10.1021/acs.jpca.6b12290
|
[12] |
X. Wang, Y. Yu, Z. Zhou, Y. Liu, Y. Yang, J. Xu, and J. Chen, J. Phys. Chem. B 123, 5782 (2019). doi: 10.1021/acs.jpcb.9b04361
|
[13] |
Z. Zhou, X. Wang, J. Chen, and J. Xu, Phys. Chem. Chem. Phys. 21, 6878 (2019). doi: 10.1039/C9CP00325H
|
[14] |
J. Eisinger and A. A. Lamola, Biochim. Biophys. Acta 240, 299 (1971). doi: 10.1016/0005-2787(71)90523-5
|
[15] |
M. E. Casida, C. Jamorski, K. C. Casida, and D. R. Salahub, J. Chem. Phys. 108, 4439 (1998). doi: 10.1063/1.475855
|
[16] |
S. Becker, C. Braatz, J. Lindner, and E. Tiemann, Chem. Phys. Lett. 208, 15 (1993). doi: 10.1016/0009-2614(93)80069-2
|
[17] |
T. Clark, J. Chandrasekhar, G. W. Spitznagel, and P. V. R. Schleyer, J. Comput. Chem. 4, 294 (1983). doi: 10.1002/jcc.540040303
|
[18] |
A. V. Marenich, C. J. Cramer, and D. G. Truhlar, J. Phys. Chem. B 113, 6378 (2009). doi: 10.1021/jp810292n
|
[19] |
L. Xiao, A. Pöthig, and L. Hintermann, Monatsh. Chem. 146, 1529 (2015). doi: 10.1007/s00706-015-1515-7
|
[20] |
J. J. Snellenburg, S. P. Laptenok, R. Seger, K. M. Mullen, and I. H. M. van Stokkum, J. Stat. Softw. 49, 1 (2012).
|
[21] |
D. Zhong, A. Douhal, and A. H. Zewail, Proc. Natl. Acad. Sci. USA 97, 14056 (2000). doi: 10.1073/pnas.250491297
|
[22] |
P. M. Hare, C. E. Crespo-Hernández, and B. Kohler, Proc. Natl. Acad. Sci. USA 104, 435 (2007). doi: 10.1073/pnas.0608055104
|
[23] |
M. M. Brister and C. E. Crespo-Hernández, J. Phys. Chem. Lett. 10, 2156 (2019). doi: 10.1021/acs.jpclett.9b00492
|
[24] |
L. Fu, Z. Wang, Y. Liu, X. Wang, R. Xu, W. Liu, J. Chen, and J. Xu, J. Photochem. Photobiol. A 396, 112491 (2020). doi: 10.1016/j.jphotochem.2020.112491
|
[25] |
K. Zhang, F. Wang, Y. Jiang, X. Wang, H. Pan, Z. Sun, H. Sun, J. Xu, and J. Chen, J. Phys. Chem. B 125, 2042 (2021). doi: 10.1021/acs.jpcb.0c10611
|
[26] |
Y. Mercier, F. Santoro, M. Reguero, and R. Improta, J. Phys. Chem. B 112, 10769 (2008). doi: 10.1021/jp804785p
|
[27] |
B. Heinz, B. Schmidt, C. Root, H. Satzger, F. Milota, B. Fierz, T. Kiefhaber, W. Zinth, and P. Gilch, Phys. Chem. Chem. Phys. 8, 3432 (2006). doi: 10.1039/b603560d
|
[28] |
R. Mundt, T. Villnow, C. T. Ziegenbein, P. Gilch, C. Marian, and V. Rai-Constapel, Phys. Chem. Chem. Phys. 18, 6637 (2016). doi: 10.1039/C5CP06849E
|
[29] |
V. Rai-Constapel, M. Etinski, and C. M. Marian, J. Phys. Chem. A 117, 3935 (2013).
|
[30] |
V. Rai-Constapel and C. M. Marian, RSC Adv. 6, 18530 (2016). doi: 10.1039/C5RA27580F
|
[31] |
T. Villnow, G. Ryseck, V. Rai-Constapel, C. M. Marian, and P. Gilch, J. Phys. Chem. A 118, 11696 (2014).
|
[32] |
M. Lv, X. Wang, H. Pan, and J. Chen, J. Phys. Chem. B 125, 13291 (2021). doi: 10.1021/acs.jpcb.1c08844
|
[1] | Yan-jun Min, Wen-peng Yuan, Dong-yuan Yang, Dong-xu Dai, Sheng-rui Yu, Guo-rong Wu, Xue-ming Yang. Ultrafast Decay Dynamics of 2-Hydroxypyridine Excited to S1 Electronic State[J]. Chinese Journal of Chemical Physics , 2022, 35(2): 242-248. DOI: 10.1063/1674-0068/cjcp2111255 |
[2] | Wen-peng Yuan, Bai-hui Feng, Dong-yuan Yang, Yan-jun Min, Sheng-rui Yu, Guo-rong Wu, Xue-ming Yang. Ultrafast Decay Dynamics of N-Ethylpyrrole Excited to the S1 Electronic State: A Femtosecond Time-Resolved Photoelectron Imaging Study[J]. Chinese Journal of Chemical Physics , 2021, 34(4): 386-392. DOI: 10.1063/1674-0068/cjcp2104060 |
[3] | Jian Li, Hai-sheng Ren, Jian-yi Ma, Xiang-yuan Li. Spectral Shift of π-π* Transition for p-Nitroaniline Based on a New Expression of Nonequilibrium Solvation Energy (cited: 1)[J]. Chinese Journal of Chemical Physics , 2014, 27(2): 233-239. DOI: 10.1063/1674-0068/27/02/181-188 |
[4] | Sheng Pan, Jia-Dan Xue, Xu-ming Zheng. Structural Dynamics of 3-Dimethylamino-2-methyl-propenal in S2(ππ*)State (cited: 3)[J]. Chinese Journal of Chemical Physics , 2014, 27(2): 149-158. DOI: 10.1063/1674-0068/27/02/149-158 |
[5] | Ya-ping Wang, Song Zhang, Si-mei Sun, Kai Liu, Bing Zhang. Ultrafast Excited State Dynamics of trans-4-Aminoazobenzene Studied by Femtosecond Transient Absorption Spectroscopy (cited: 1)[J]. Chinese Journal of Chemical Physics , 2013, 26(6): 651-655. DOI: 10.1063/1674-0068/26/06/651-655 |
[6] | Xiao-lan Song¤, Xi He, Hai-ping Yang, Yi-xin Qu, Guan-zhou Qiu. Grain Growth Kinetics of BaTiO3 Nanocrystals During Calcining Process[J]. Chinese Journal of Chemical Physics , 2008, 21(3): 295-300. DOI: 10.1088/1674-0068/21/03/295-300 |
[7] | Yuan-zuo Li, Yu Sun, Yong-qing Li, Feng-cai Ma. Electronic Structure and Photophysical Properties of 2-(N,N-diethylanilin-4-yl)-4,6-bis(3,5-dimethylpyrazol-1-yl)-1,3,5-triazine[J]. Chinese Journal of Chemical Physics , 2007, 20(1): 59-64. DOI: 10.1360/cjcp2007.20(1).59.6 |
[8] | Quan Li, Fang-qian Huang, Jing-dan Hu, Ke-qing Zhao. Hydrogen-bonding Interaction of 1,2,3-triazine-waters Complexes[J]. Chinese Journal of Chemical Physics , 2006, 19(5): 401-405. DOI: 10.1360/cjcp2006.19(5).401.5 |
[9] | Xu Jianhua, Hu Wuhong. A Quantum Chemistry Study on the Isomerization Reaction Mechanism of ATM[J]. Chinese Journal of Chemical Physics , 2003, 16(3): 189-192. DOI: 10.1088/1674-0068/16/3/189-192 |
[10] | Huang Xin, Liu Lei, Li Xiaosong, Guo Qingxiang, Liu Youcheng. A PM3 Molecular Orbital Study on the Conformational Equilibrium of Cyclohexane upon α-Cyclodextrin Inclusion Complexation[J]. Chinese Journal of Chemical Physics , 2000, 13(5): 539-543. DOI: 10.1088/1674-0068/13/5/539-543 |
1. | Zhou, J., Wang, X., Jia, M. et al. Ultrafast spectroscopy study of DNA photophysics after proflavine intercalation. Journal of Chemical Physics, 2024, 160(12): 124305. DOI:10.1063/5.0194608 |
2. | Hu, K., He, X., Jin, P. et al. Unravelling the Competition between Internal Conversion and Intersystem Crossing in Twisted molecule 9-Phenylacridine by Femtosecond Time-resolved Spectroscopy. ChemPhotoChem, 2024. DOI:10.1002/cptc.202400108 |
3. | Wu, P., Wang, X., Pan, H. et al. Wavelength dependent excited state dynamics observed in canonical pyrimidine nucleosides. Journal of Photochemistry and Photobiology, 2023. DOI:10.1016/j.jpap.2023.100211 |
4. | Han, Y., Wang, X., He, X. et al. Excited State Kinetics of Benzo[a]pyrene Is Affected by Oxygen and DNA. Molecules, 2023, 28(13): 5269. DOI:10.3390/molecules28135269 |
5. | Wang, D., Wang, X., Jin, P. et al. Distinct doorway states lead to triplet excited state generation in epigenetic RNA nucleosides. Physical Chemistry Chemical Physics, 2023. DOI:10.1039/d3cp00772c |
6. | Zhou, J., Jia, Y., Wang, X. et al. Excited-State Dynamics of Proflavine after Intercalation into DNA Duplex. Molecules, 2022, 27(23): 8157. DOI:10.3390/molecules27238157 |
![]() |
![]() |
![]() |